Background
Acoustic imaging is interesting because of its ability to detect and image objects through optically opaque and inhomogeneous media, as is done when imaging babies in utero with ultrasound. The basic principles of this sensing technique have been understood since the advent of active sonar systems in the second World War. Advancement of the technology was relatively slow until the development of digital signal processing and the associated computing capabilities, after which there was an explosion of growth in signal processing for SONAR and other acoustical signal processing applications, mainly medical ultrasound and seismic exploration for oil and gas. In the last decade, theory and experiment have developed to the point where imaging is now possible even in scattering, inhomogeneous media like the human body. We were interested in seeing whether or not the technology has evolved to the point where it can be applied to constructing an image through or around walls.
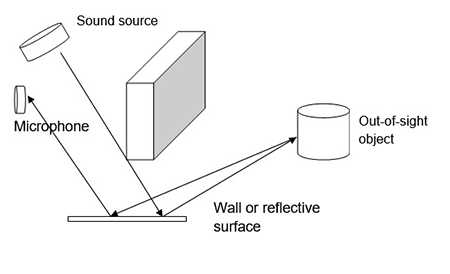
Figure 1: Proposed experimental configuration.
Recent work by Lindell demonstrated a technique for reconstructing a simple scene consisting of large planar shapes against a distant background. We were motivated by that work to consider how it would work with obstructions, like a wall or a door. The published work also overlooked the benefits of using higher frequency sound, namely that it is inaudible and can produce higher resolution images. We imagined experiments with a configuration shown in Figure 1.
Approach
We set up a system with reflective targets similar to that shown in Figure 1 except that we started without an intervening wall or door. For the sensors, we used a fixed speaker and inexpensive MEMS microphones in a linear 16 element microphone array attached to a linear scanning stage. We used a combination of simple target objects, including a dinner plate, a ball, a plywood sheet, etc., all in various positions. Due to hardware difficulties (out-of-spec microphones and linear stage making ultrasonic noises) we were unable to complete the data acquisition for all targets, and only collected reflections from the plywood sheet.
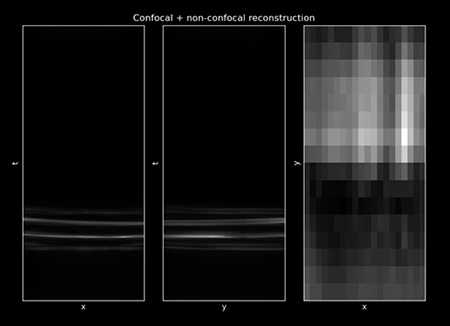
Figure 2: Our reconstruction of an erect plywood sheet using the reflected sound data. This was done using the light cone transform.
Accomplishments
The light cone transform of Lindell, et. al. was implemented for the reconstruction of the image of the hidden plywood sheet, and the result is shown in Figure 2. We achieved an upper frequency limit of 40 kHz with our data acquisition hardware, demonstrating that ultrasonic operation is possible. Improvements to the system that could be done include the calibration of the microphones for flat frequency response and mounting the translation stage power supply in a soundproof box so that its ultrasonic sounds don’t interfere with the imaging process.