Click below to jump to an article of interest.
Fabrication Facilities

SwRI develops custom components and fixtures to support advanced science and applied engineering programs. From micro-sized parts to large integrated systems, our Mechanical Fabrication Center ensures that components meet America Society of Mechanical Engineers and other specifications with equipment calibrated and traceable to the Bureau of Standards.
State-of-the-art fabrication capabilities support:
- Machining exotic materials, such as alloys, titanium, Al/AlO2 and ceramics
- Microcomponents to parts 36 inches in diameter
- Large and small milling operations
- Computer numerical controlled (CNC) turning and machining
- Computer-aided design (CAD) production
- Welding with tungsten inert gas (TIG), metal inert gas (MIG), shielded metal arc (SMAW) and oxy-acetylene
For more information, visit Mechanical Fabrication Center.
SwRI Tapped for IMAP Mission

SwRI is developing the Compact Dual Ion Composition Experiment (pictured below) for IMAP. CoDICE is a novel sensor combining multiple capabilities into one patented sensor about the size of a 5-gallon paint bucket and weighing about 22 pounds.
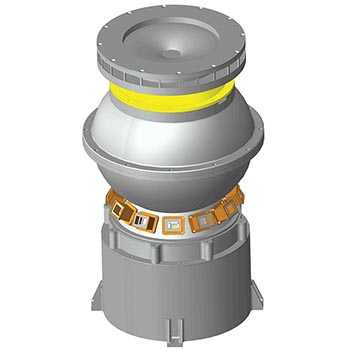
SwRI will manage the payload and payload systems engineering for a new NASA mission that will sample, analyze and map particles streaming to Earth from interstellar space. SwRI also will provide a scientific instrument and other technology for the Interstellar Mapping and Acceleration Probe (IMAP) spacecraft, scheduled to launch in 2024.
IMAP will help researchers better understand the boundary of the heliosphere, a sort of magnetic bubble surrounding and protecting our solar system. This is where the constant flow of particles from the Sun, called the solar wind, collides with material from the rest of the galaxy. This bubble limits the amount of harmful cosmic radiation entering the heliosphere. IMAP instruments will collect and analyze particles that make it through.
“SwRI plays a major hardware role in the mission, which will allow a quantum leap forward in our understanding of our heliosphere’s interaction with, and our place in, the galaxy,” said SwRI Director Susan Pope, who will serve as the payload systems engineer.
“We’re also providing the Compact Dual Ion Composition Experiment (CoDICE), which was developed using SwRI internal research funding,” said Dr. Mihir Desai, director of the SwRI Space Research Department and an IMAP co-investigator. “CoDICE combines the capabilities of multiple instruments into one patented sensor about the size of a 5-gallon paint bucket and weighing about 22 pounds.”
It will measure the distributions and mass, ionic charge state and composition of interstellar pickup ions, particles that make it through the “heliospheric” filter. It also characterizes solar wind ions as well as the mass and composition of highly energized particles from the Sun.
SwRI is also contributing to the development of next-generation energetic neutral atom imagers, as well as electronics for the IMAP instruments that measure solar wind electrons.
“I am looking forward to IMAP, which will help explain the discoveries that the IBEX mission is still making,” said SwRI Program Director John Scherrer, who will serve as the payload manager. “IMAP will help us understand how our Sun and the solar wind affect the boundary of our solar system.”
The IMAP mission principal investigator is former SwRI staff member Prof. David McComas of Princeton University. The Johns Hopkins University Applied Physics Laboratory in Laurel, Maryland, manages IMAP and is designing, building and will operate the spacecraft. The mission is part of the NASA Science Mission Directorate’s Solar Terrestrial Probes Program, managed by Goddard Space Flight Center in Greenbelt, Maryland.
For more information, visit Heliophysics.
Super-Hydrophobic Coating
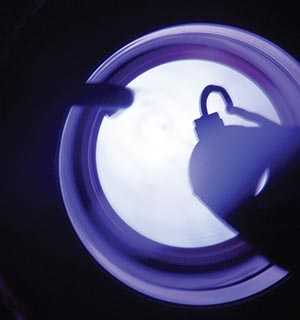
The novel process creates a plasma coating along the length of the pipe.

SwRI developed a vacuum process to produce superhydrophobic coatings on internal pipe surfaces. These thin, glass-like coatings are durable and repel deposits that can block oil and gas production lines.
SwRI has developed a superhydrophobic — or water-repelling — coating and a process for applying it to internal surfaces of long tubular structures. The coatings passively prevent hydrates and other deposits from adhering to subsea pipelines and clogging oil and gas transmission lines.
“The Lotus coating technology is named for the flower that has similar superhydrophobic, water repelling properties,” said Institute Scientist Dr. Michael Miller. “We produce these coatings using a vacuum process in which a plasma — a state of matter with free electrons and ionized atoms and molecules — is ignited inside the entire length of the pipe while introducing one or more chemicals. The process selectively fragments the chemicals, accelerating the ions, which adhere to the pipe surface. There, they immediately undergo polymerization to form a thin, glass-like, durable coating that repels water and deposits.”

A water droplet on the inside surface of a coated pipe illustrates the hydrophobicity of the coating.
SwRI’s Lotus production process can be tailored chemically to create different surface properties, such as surface energy or tension, to repel different kinds of deposit materials.
For instance, hydrates are slushy solids composed of water and a gas that have a density close to that of ice but form at temperatures as high as 70 degrees Fahrenheit. In hydrocarbon production lines, hydrates can adhere to a pipe’s inner wall and build until they completely block the flow line and halt the transport of hydrocarbons to the processing facility. Similarly, in deep petroleum wells, paraffin (wax) and asphaltene components can create pipeline deposits and impede product flow from the well. Removing these pipeline plugs is time-consuming and costly, so a passive prevention technique is ideal.
SwRI has licensed the Lotus coating technology exclusively to its client, which is transitioning the process for full-scale production of coated pipes.
For more information, visit Surface Engineering & Materials Chemistry Facilities.
Finding Water in Moonshine
Using uncommon expertise in how water reacts with lunar soil, an SwRI scientist contributed to studies indicating that water and/or hydroxyl may be more prevalent on the Moon’s surface than previously thought.
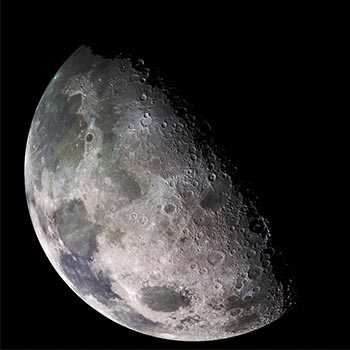
“Water on the Moon is of intense interest for many reasons,” said SwRI’s Dr. Michael J. Poston, a coauthor of the paper, “Widespread Distribution of OH/H2O on the Lunar Surface Inferred from Spectral Data,” published in Nature Geoscience online. Water has been the focus of many lunar missions, largely because it is a critical resource for a Moon habitat. “When you split water molecules, you end up with oxygen and hydrogen, critical components for breathable air and rocket fuel. Hydroxyl (OH) is also useful, but takes more energy to access and provides less hydrogen, making it less attractive.”
To understand how tightly water is bound to lunar surface materials, Poston conducted extensive experiments with water and lunar samples collected by the Apollo missions in the late 1960s and early 1970s. Using detailed surface temperature maps and thermophysics modeling, the team estimated the amount of Moon glow to subtract from the reflected sunlight to better characterize the inferred measurements of water on the lunar surface. Based on the results, it appears that OH/H2O is present on lunar surfaces under much more wide-ranging conditions than previously understood.Up until the past decade or so, scientists thought the Moon was arid, with water existing perhaps only as ice in the permanently shaded craters near the poles. In the late 2000s, however, analysis of lunar samples and data from new spacecraft instruments showed evidence to the contrary.
“The next step is to determine whether it’s water, hydroxyl, or a mixture of the two — and where it came from,” Poston said.
Putting Regenerative Medicine into Production
SwRI has joined the Advanced Regenerative Manufacturing Institute (ARMI) to help advance large-scale manufacturing of engineered tissues and related technology. ARMI is a consortium of nearly 100 organizations dedicated to developing next-generation manufacturing processes and technologies for cells, tissues and organs.
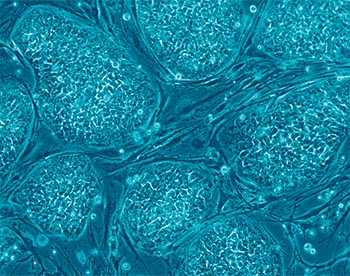
“The next frontier of regenerative medicine is manufacturing,” said Dr. Jian Ling, an Institute scientist in the Chemistry and Chemical Engineering Division. “By joining ARMI, SwRI can work with other members to transition regenerative medicine technologies to manufacturing and bring significant additional benefits to patients. SwRI has the experience and resources, especially in product development and technology commercialization. Through the consortium, we can collaborate with other members and accelerate transition of our technology.”
SwRI expertise aligns well with ARMI’s strengths. Our staff has extensive experience in regenerative medicine, including engineering skin, bone, tendon and blood vessel materials for novel wound-healing applications. SwRI offers bioreactors to scale-up stem cell production and facilities to encapsulate cells, growth factors and drugs for bioprint applications.
“ARMI membership allows SwRI to be at the cutting edge of regenerative medicine when R&D is beginning to transition out of the lab into large-scale commercial operations,” said SwRI Vice President Dr. Michael MacNaughton. “SwRI chemists can collaborate with our robotics, automation, modeling and simulation experts to improve manufacturing processes, data analysis and control software.”
In addition to CGMP laboratories for pilot production, SwRI offers an established ISO 13485 Quality System for medical device design, prototyping and testing.
According to ARMI, approximately $80 million in federal funds awarded by the Department of Defense will be combined with more than $200 million in cost-sharing investments to support the development of tissue and organ manufacturing capabilities.
For more information, visit Biomaterials & Scaffolds.
Adapting Drones to Inspect Nuclear Power Plants

The team developed an enclosed obstacle course to simulate potential conditions inside the containment at Fukushima Daiichi. The photo on the top shows the UAS autonomously traveling around obstacles in the test fixture. The image on the bottom depicts the original (purple line), actual (blue circles), and upcoming (green circles) flight paths as the UAS travels around the obstacles (red blocks).

An SwRI-led team is developing unmanned aerial system (UAS) technology to autonomously fly into the containment vessels of the damaged units at Japan’s Fukushima Daiichi nuclear power station and assess conditions.
SwRI teamed with the General Robotics, Automation, Sensing and Perception (GRASP) Lab at the University of Pennsylvania (Penn) to successfully demonstrate the feasibility of the approach in a test fixture at SwRI. The team also verified that the UAS components could survive the harsh radiation conditions within the containment.
“The conditions inside the containment at Fukushima Daiichi are quite possibly the most challenging environment that the SwRI–Penn team has had to address,” said Project Manager Dr. Monica Garcia of SwRI’s Intelligent Systems Division. “We will be pushing the envelope in terms of the technology.”
When a 9.0 magnitude earthquake and a 13-meter-high tsunami struck the power station in 2011, this one-two punch initiated a series of events that ultimately caused three reactors to fail. Since then, a number of ground- and underwater-based robotic systems have been sent inside the containment to investigate. However, damage and high radiation levels have limited access to information vital to decontamination and decommissioning efforts.
“The team is adapting high-speed, advanced mobility drones to collect key information about the current status,” said Dr. Richard Garcia, SwRI’s technical lead. “This information will play an important role in future decontamination and decommission efforts at Fukushima Daiichi.”
“As robots get smaller, faster and smarter, this is exactly the kind of problem we want them to address,” said Dr. Vijay Kumar, the Nemirovsky Family Dean of Penn’s School of Engineering and Applied Science. “Challenges like this are what push research in our field forward.”
Tokyo Electric Power Company Holdings Inc. (TEPCO Holdings) contracted SwRI to explore the use of UASs, or drones, within the containment at Fukushima Daiichi. Working with Penn’s GRASP Lab, SwRI engineers are helping adapt small drones to autonomously operate in this challenging environment.
For more information, visit Unmanned Aerial Systems.
Fatigue Failure Prevention
SwRI’s 25th NASGRO® short course at our San Antonio headquarters will be presented on October 23-25, 2018. The last five classes for the fatigue and fracture analysis tool have sold out.
NASGRO, the most widely used fracture mechanics and fatigue crack growth software in the world, is used to evaluate structural components of aircraft, spacecraft, rotorcraft, gas turbine engines, pressure vessels, aging infrastructure and more.
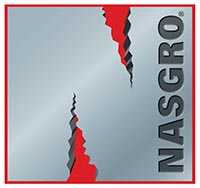
“NASGRO is a suite of computer programs to analyze fracture and fatigue crack growth in structures and mechanical components,” said Dr. Craig McClung, who along with Joe Cardinal has also traveled the world teaching these short courses at client locations. “In more than 60 total courses, SwRI has trained more than 1,100 people to evaluate and demonstrate that aircraft, spacecraft, rotorcraft and gas turbine engines are safe for flight.”
Developed by SwRI engineers and NASA under a Space Act Agreement, NASGRO is also supported by the NASGRO Consortium and the Federal Aviation Administration. NASGRO’s integrated modules calculate stress intensity factors, compute crack growth, and determine critical crack sizes. The software stores fatigue crack growth and fracture toughness data in an extensive material property database enabling the evaluation of many different types of materials and structures.
To register for the NASGRO training, go to swri.org/nasgro-training.
For more information on NASGRO and the NASGRO Consortium, visit nasgro.swri.org.
Diverting Lava Flows
As Hawaii’s Kilauea Volcano continues to wreak havoc on the Big Island, SwRI engineers remember a 1970s-era project to investigate using water-filled metal vessels to divert lava flows threatening civilization.
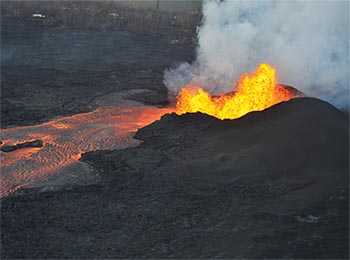
Engineers conducted an “Initial Feasibility Study of Water Vessels for Arresting Lava Flow” for the U.S. Army Material Systems Analysis Activity. Using analysis and experiments, they investigated small cylinders filled with water instead of explosives that had been tried in the past. These vessels contain water that is transformed into high-pressure, superheated steam when exposed to the extreme temperatures of molten lava. While experiments showed the ruptured water vessels could be energetic enough to disturb flows, the development never quite made it out of the lab. Hawaiians rejected field testing the technology, thinking it would offend Pele, the island’s volcano goddess.
The thousands devastated by Pele’s latest round of activity might wish that SwRI had progressed past this initial study. However, measures to stop lava flows around the world have had limited success. When it’s man versus volcano — or Pele — the volcano ultimately wins.
Cosmochemical Model of Pluto Formation
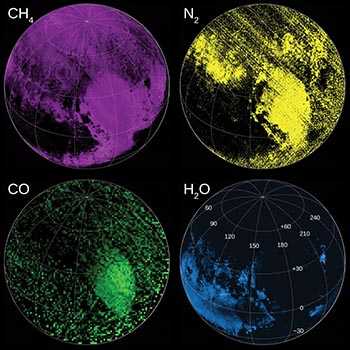
These maps — assembled from New Horizons’ Ralph instrument — indicate regions rich in methane (CH4), nitrogen (N2), carbon monoxide (CO) and water (H2O) ices. Sputnik Planitia shows an especially strong signature of nitrogen near the equator. SwRI scientists combined these data with Rosetta’s comet 67P data to develop a proposed cosmochemical model for Pluto formation.
SwRI scientists integrated NASA’s New Horizons discoveries with data from ESA’s Rosetta mission to develop a new theory about how Pluto may have formed at the edge of our solar system.
“We’ve developed a cosmochemical model of Pluto formation,” said Dr. Christopher Glein. The research is described in a paper published in Icarus. At the heart of the research is the nitrogen-rich ice in Sputnik Planitia, a large glacier that forms the left lobe of the bright Tombaugh Regio feature on Pluto’s surface.
“We found an intriguing consistency between the estimated amount of nitrogen inside the glacier and the amount that would be expected if Pluto was formed by the agglomeration of roughly a billion comets or other small Kuiper Belt Objects similar in chemical composition to 67P, the comet explored by Rosetta,” Glein said.
Scientists needed to understand not only the nitrogen present at Pluto now — in its atmosphere and in glaciers — but also how much of the volatile element potentially could have leaked out of the atmosphere and into space over the eons. They then needed to reconcile the proportion of carbon monoxide to nitrogen to get a more complete picture. Ultimately, the low abundance of carbon monoxide at Pluto points to burial in surface ices or to destruction from liquid water.
“Our research suggests that Pluto’s initial chemical makeup, inherited from cometary building blocks, was chemically modified by liquid water, perhaps even in a subsurface ocean,” Glein said. However, a solar model also satisfies some constraints. While the research pointed to some interesting possibilities, many questions remain to be answered.
“This research builds upon the fantastic successes of the New Horizons and Rosetta missions to expand our understanding of the origin and evolution of Pluto,” said Glein. “Using chemistry as a detective’s tool, we are able to trace certain features we see on Pluto today to formation processes from long ago. This leads to a new appreciation of the richness of Pluto’s ‘life story,’ which we are only starting to grasp.”
For more information, visit Planetary Science.
Nickname for Ultimate Target
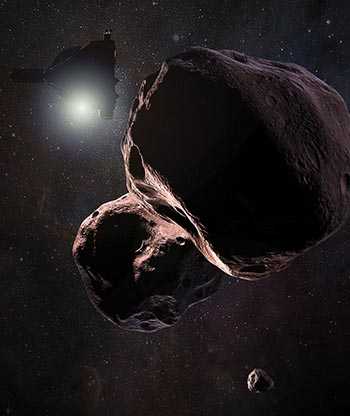
This illustration depicts NASA’s New Horizons spacecraft encountering 2014 MU69, aka Ultima Thule, a Kuiper Belt object that orbits the Sun a billion miles beyond Pluto.
As NASA’s New Horizons spacecraft continues exploring the unknown, the SwRI-led mission team has selected a nickname for the Kuiper Belt Object (KBO) it will visit on Jan. 1, 2019.
After being nominated and voted on by the public, the name “Ultima Thule” (pronounced ultima too-lee) was chosen for the spacecraft’s next target at the edge of the solar system. Officially known as 2014 MU69, the KBO will be the most primitive world ever explored, and the farthest exploration of any world in history — a billion miles beyond Pluto.
In ancient literature, Thule is a remote mythical island referenced as the “ends of the Earth.” Ultima Thule means beyond Thule, beyond the borders of the known world. This moniker epitomizes New Horizons’ journey to a distant place never visited before.
“MU69 is humanity’s next Ultima Thule,” said SwRI’s Dr. Alan Stern, New Horizons principal investigator. “Our spacecraft is heading beyond the limits of the known worlds, to what will be this mission’s next achievement. Since this will be the farthest exploration of any object in space in history, I like to call our flyby target Ultima, for short, symbolizing this stage of ultimate exploration by NASA and our team.”
After the flyby, NASA and the New Horizons team will choose a formal name to submit to the International Astronomical Union, based in part on whether MU69 is found to be a single body, a binary pair or perhaps multiple objects.
For more information, visit Planetary Science.
Advancing Energy Technology
The U.S. Department of Energy has awarded SwRI three contracts worth more than $2 million to advance turbine technology for power plants.
“These projects are a part of SwRI’s continuing contribution toward DOE’s goal of developing the most efficient and reliable energy infrastructure,” said Dr. Klaus Brun, a program director in SwRI’s Mechanical Engineering Division.
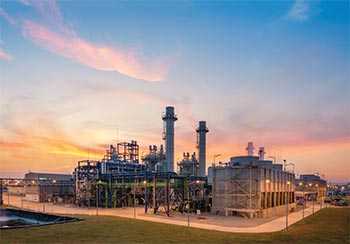
In one effort, SwRI will develop a concept for a supercritical carbon dioxide (sCO2), coal syngas or natural gas-fired oxy-fuel turbine. The goal is to improve high-cycle efficiencies and increase power output, thus reducing both the size and cost of a power plant. SwRI will lead a team in this effort.
For the second project, SwRI will develop a modular, highly efficient combined-cycle power system. The system will be cleaner and more fuel-efficient, reducing operating costs and plant size. In addition, an sCO2-based waste-heat recovery system will be added to an existing gas turbine to increase efficiency and environmental performance. This effort is also led by SwRI and includes several collaborators.
A third project is developing coal-fired flameless pressurized oxy-combustion technology for a 50-megawatt pilot power plant. The project has three phases. The first phase seeks to reduce pilot plant costs, begin an environmental impact study and secure a host site for a pilot plant. Engineers also will work with collaborators to develop an advanced turbo-expander that will extract extra power from flue gases.
SwRI is a leader in research and development of oxy-combustion cycle technology, which aims to provide high-efficiency electricity generation for next-generation power plants.
For more information, visit Conventional Power Generation.
Flash Space Storage
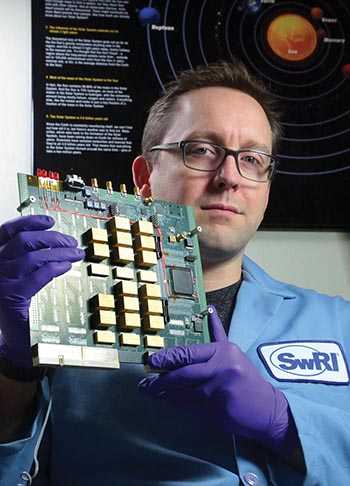
SwRI engineers patented a flash memory storage system that allows satellites to collect and store vast amounts of data for later transmission to ground stations.
“The technology provides high-speed, high-capacity storage of satellite data,” said Michael Koets, a staff engineer in the Space Science and Engineering Division. “A representative application is storing weather satellite data.”
Weather satellites rapidly generate high volumes of high-resolution images. However, the satellites can only download data to receivers on the ground in certain locations.
“All that data needs to be stored to memory on the satellite as it is collected,” Koets said. “Our system can record data to flash memory up to 100 times faster than previous approaches.”
Flash memory is efficient and compact, allowing smaller, less expensive satellites to gather much more scientific data than conventional data recording devices. Flash memory is ubiquitous in consumer electronics such as mobile phones and thumb drives, but the rigors of the space environment have slowed its adoption for satellites and interplanetary spacecraft. The SwRI technology allows the use of slower but more robust flash memories that can operate in space while achieving performance close to that of contemporary consumer electronics.
Other potential applications include storing radar data collected by Earth- monitoring satellites studying glaciers or earthquake zones, or storing scientific data collected by deep space missions, such as those sent to investigate asteroids.
The patented technology was developed by Koets, Larry T. McDaniel III and (at right) Miles R. Darnell.
For more information, visit Space Avionics Systems.
CNWRA Contract Renewed
The U.S. Nuclear Regulatory Commission (NRC) has renewed its contract with SwRI to operate the Center for Nuclear Waste Regulatory Analyses (CNWRA).
The contract is valued at up to $52 million over a five-year period of performance, including a one-year base period and four one-year option periods. Under this contract, CNWRA continues to provide technical assistance and research support to NRC activities related to storage, transportation, possible reprocessing and ultimate geological disposal of spent nuclear fuel and high-level radioactive wastes.
“For 30 years, CNWRA has been central to supporting NRC’s mission through evaluations of engineering and environmental and scientific factors affecting management of radioactive wastes,” said Dr. Wesley C. Patrick, CNWRA executive director.

Recognized as a center of excellence in earth science and engineering, CNWRA supports the NRC with a broad range of technical assistance and research. CNWRA expertise includes environmental evaluations, fire protection engineering, hazard assessments, materials degradation and aging management. The center provides performance and probabilistic risk assessments, risk-informed license review and site characterization. It also supports public outreach and stakeholder engagement associated with rule-making and licensing activities.
“SwRI is proud that our CNWRA operations support the NRC’s mission to protect public health and safety,” said SwRI President and CEO Adam Hamilton. “Our staff members have expertise spanning the environmental, geological and material sciences, as well as the engineering disciplines needed to evaluate safety and environmental compliance of nuclear facilities.”
With facilities in San Antonio and Rockville, Maryland, CNWRA also conducts independent research and peer reviews related to radioactive waste management for foreign governments and regulatory agencies around the world.
For more information, visit CNWRA.
Martian Moon Models
SwRI scientists believe Martian moons formed following a cosmic collision, but on a much smaller scale than the giant impact thought to have resulted in the Earth-Moon system. Their work shows that an impact between proto-Mars and a dwarf-planet-sized object likely produced Deimos and Phobos, as detailed in a paper published in Science Advances.
The origin of the Red Planet’s small moons has been debated for decades. The question is whether the bodies were asteroids captured intact by Mars’ gravity or whether the tiny satellites formed from an equatorial disk of debris, as is most consistent with their nearly circular and coplanar orbits.
“Ours is the first self-consistent model to identify the type of impact needed to lead to the formation of Mars’ two small moons,” said SwRI’s Dr. Robin Canup, one of the leading scientists using large-scale hydrodynamical simulations to model planet-scale collisions, including the prevailing Earth-Moon formation model.
“A key result of the new work is the size of the impactor,” Canup said. “ We find that a large impactor — similar in size to the largest asteroids — is needed, rather than a giant impactor.”
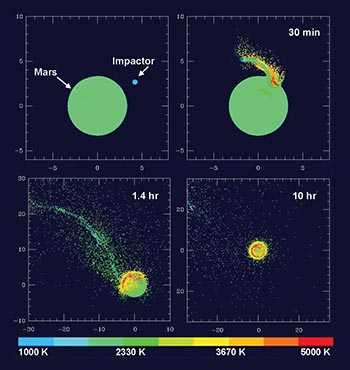
Deimos and Phobos are very small, with diameters of only 7.5 miles and 14 miles respectively, and orbit very close to Mars. The proposed Phobos-Deimos forming impactor would be between the size of the asteroid Vesta, which has a diameter of 326 miles, and the dwarf planet Ceres, which is 587 miles wide.
“Our state-of-the-art models show that a Vesta-to-Ceres-sized impactor can produce a disk consistent with the formation of Mars’ small moons,” said the paper’s coauthor, Dr. Julien Salmon. “The outer portions of the disk accumulate into Phobos and Deimos, while the inner portions of the disk accumulate into larger moons that eventually spiral inward and are assimilated into Mars.”
The research was funded by NASA’s Solar System Exploration Research Virtual Institute (SSERVI) in Silicon Valley, and by NASA’s Emerging Worlds program. The research was conducted as part of the Institute for the Science of Exploration Targets (ISET), a SSERVI team from SwRI’s Boulder, Colorado, branch.
For more information, visit Planetary Science.
Propellant Delivery
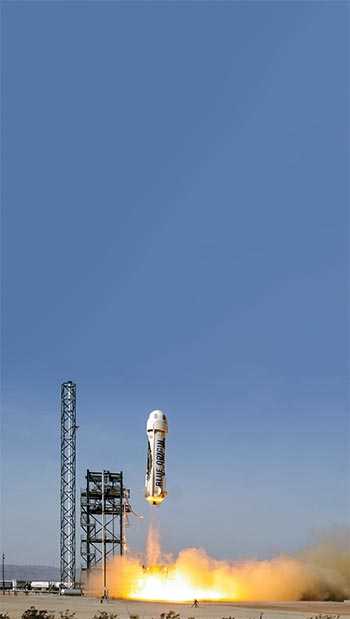
NASA has tapped SwRI to test new technology to more efficiently and reliably deliver liquid propellants for future space missions. SwRI and NASA’s Glenn Research Center developed the tapered liquid acquisition device (LAD), which will be evaluated in microgravity.
The device is designed to deliver cryogenic liquid propellants to a rocket engine from fuel tanks. The tapered LAD also can transfer fuel between tanks. Existing LAD designs with straight channels generate vapor bubbles in the delivery channel. SwRI’s tapered design passively removes the bubbles through surface tension forces to substantially improve the transfer of cryogenic fluids at lower costs.
SwRI will adapt the ground-tested hardware for an autonomous experiment that will fly on New Shepard, Blue Origin’s reusable launch vehicle.
“New Shepard provides approximately three minutes of high-quality microgravity, which is significant when compared to the 25 seconds of microgravity achieved in parabolic flights,” said Kevin Supak, who is leading the project. “Once flight testing in microgravity is completed, the device potentially could be tested onboard the International Space Station in extended microgravity and eventually be incorporated into future cryogenic propellant tank designs.”
The flight test will take place early 2019 in West Texas at Blue Origin’s launch site near Van Horn. SwRI researchers will be onsite to oversee experiment preparation and view the launch.
SwRI has more than 60 years of experience studying propellant dynamics for launch vehicles and spacecraft propulsion systems.
For more information, visit Space Propulsion & Propellants and Liquid Propulsion.