From coastal towns to rural farms to urban centers, climate change threatens not just the environment, it also affects human health, community stability, national security and overall economic well-being. Climate change fuels extreme weather, from droughts and floods to tornados, wildfires and other catastrophic events that wreak havoc on our communities, institutions and the environment. To address these issues, the international community has focused on decarbonization — reducing the most prevalent greenhouse gases emitted by burning fossil fuels, clearing land and producing food and manufactured products.
This shift has resulted in significant changes to the power generation mix on global, national and local scales. Growth in energy demand has slowed in recent years, and the fuel mix has shifted from coal toward natural gas and renewables. While fossil-based sources and nuclear power still accounted for 75% of the power generated, the remaining 25% was produced by hydroelectric (15.8%), wind (4.8%), solar (2.2%) and geothermal/ biomass (2.4% combined) sources. Wind and solar sources remain a relatively low percentage of the overall energy mix, but they are the fastest-growing categories globally and particularly for Organisation for Economic Co-operation and Development (OECD) member countries, including the United States. From 2017 to 2018, the International Energy Association reported overall declines in electricity production in OECD countries from combustible fuels — particularly coal and oil — which were substantially offset by a 19.8% and 7% growth in solar and wind production, respectively.
The rapid introduction of renewable resources into the energy mix has introduced two significant challenges. First, the capacity growth provided from solar and wind generators is highly variable. Output can fluctuate significantly by the minute, hour and season. Wind speed varies with weather patterns or diurnal effects. Likewise, solar power output is affected by storms, passing clouds, ambient temperature and wind. The second challenge is the mismatch between availability of wind and solar resources and typical electrical demand profiles, leading to daily imbalances in generation and demand. This means renewable power sources must be supplemented by more traditional power plants that can take a long time to ramp up and shut down. Simple-cycle gas turbines allow fast-startup and high-turndown capabilities but with relatively poor efficiency and high emissions at low loads. Combined-cycle gas turbines and steam turbines used in large baseload fossil or nuclear plants incorporate large heat exchangers that limit ramp-rate capabilities to minimize thermal stress but with higher partial-load efficiencies and lower partial-load emissions.
ABOUT THE AUTHOR
Dr. Tim Allison is director of SwRI’s Machinery Department, which performs applied research in power systems, including advanced energy systems, thermal-mechanical-chemical energy storage, oxy-combustion, and turbomachinery design, fabrication, instrumentation, testing and control.
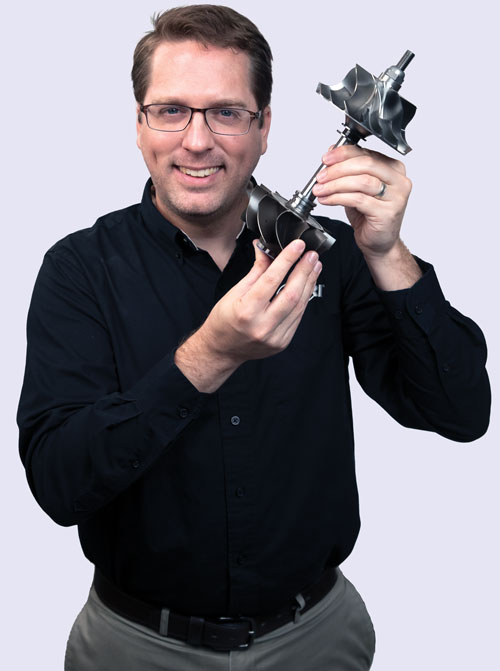
Significant global integration of renewable energy sources with high variability into the power generation mix requires new cost-effective, efficient and reliable grid-scale energy storage technologies. These systems store energy when excess renewable power is available and discharge it when renewable generation drops off or when demand exceeds supply. The many forms of energy have resulted in a wide range of technologies for energy storage, some that are commercially mature and others that are currently under development. Notably, the application space for electrochemical (usually lithium-ion) batteries has grown significantly in recent years up to the 100-plus MW scale. But these systems are still only cost-effective for up to about four hours of storage, which is inadequate for accommodating deep penetration of renewables. Longer-duration grid-scale technologies include commercially mature technologies such as pumped hydro energy storage and compressed air energy storage (CAES) as well as developing technologies that target unprecedented storage durations at low cost, including pumped heat energy storage, liquid air energy storage, hydrogen-based thermochemical storage and advancements to pumped hydro and CAES.
Southwest Research Institute is developing and advancing technologies aimed at providing cost-effective, long-duration storage for the electric grid, including projects funded by the U.S. Department of Energy and commercial collaborators. This article provides an overview of some these technology development efforts.
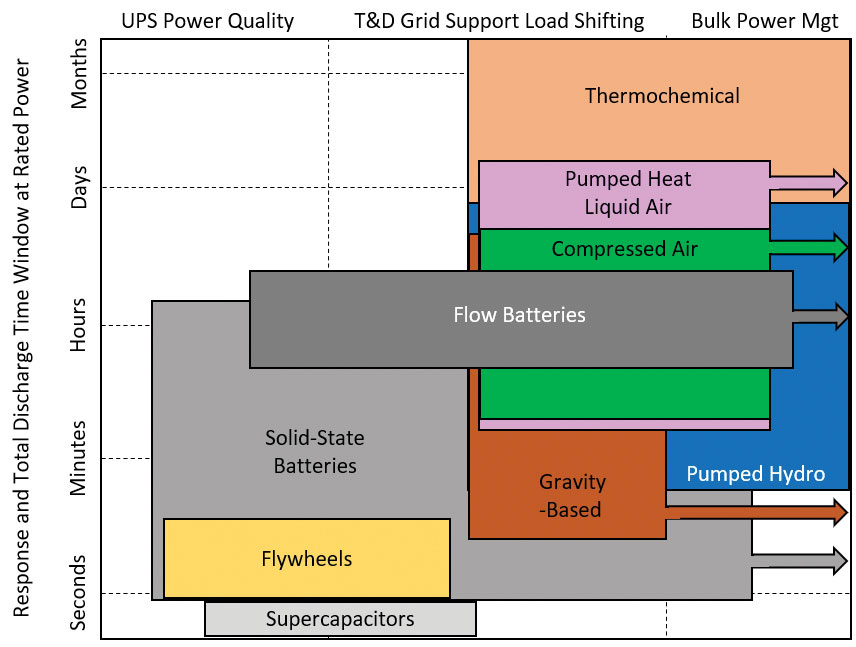
This graph illustrates mature and developing energy storage technologies, identifying nominal discharge times and operating scales for various technologies.
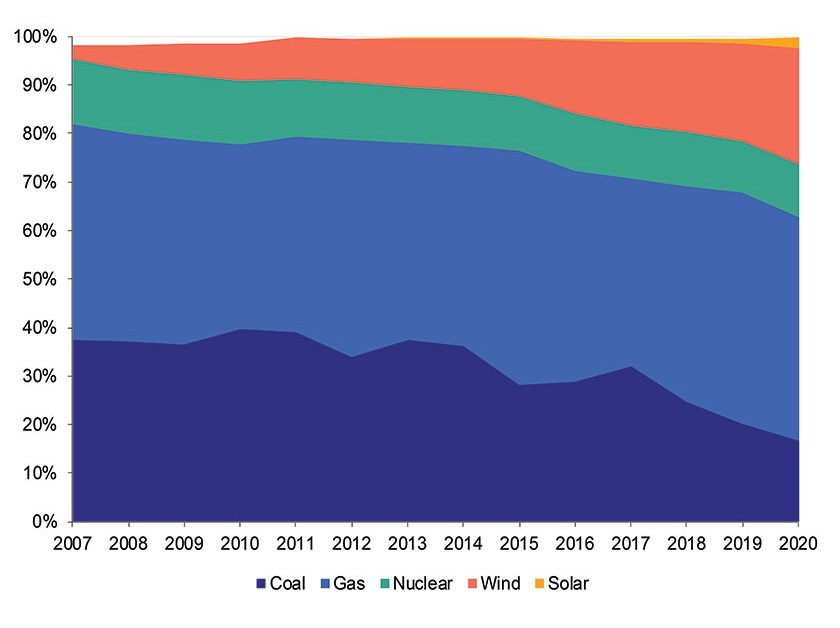
Many of SwRI’s energy storage research projects are conducted in cooperation with Texas power generators. This graph shows the historic power generation mix for ERCOT, which manages the majority of Texas’ power grid.
1. Storing Sunlight
Jason Wilkes, Ph.D.In a Department of Energy project, SwRI is helping develop machinery for a concentrated solar power (CSP) plant that combines supercritical carbon dioxide (sCO2) power cycles with integrated thermal energy storage. CSP technology uses mirrors or lenses to concentrate a large amount of sunlight onto a receiver, which typically converts concentrated light to heat and extracts thermal energy to generate power using steam turbines. Next-generation CSP systems will store the energy as heat, which can be converted to on-demand energy using sCO2 power cycles, improving efficiency and reducing operating costs. sCO2 is carbon dioxide held above a critical temperature and pressure, which causes it to act like a gas while having the density of a liquid. This state makes sCO2 a highly efficient fluid for power generation because small changes in temperature or pressure cause significant shifts in density. Utilizing sCO2 as a working fluid can increase the efficiency of a CSP plant by as much as 10 percentage points.
Additionally, integrating thermal energy storage will allow for a more stable grid. Renewable power sources such as CSP can be variable, but the ability to store excess power at peak production times will help meet peak demands. Existing CSP plants typically use thermal oil or molten nitrate salts as a storage medium at temperatures up to 565 degrees Celsius. Next-generation sCO2 plants target lower-cost solid media allowing thermal storage at higher temperatures, potentially up to 630–750 C. The sCO2 machinery design features an innovative compact skid-based arrangement with the potential for containing all turbomachinery (in this case an integrally geared compander, or compressor/expander), recuperators, control systems and ancillary hardware on a single skid. This simplifies installation by reducing the number of field welds and gas, electrical and piping connections made on site. With this approach, the project team can realize a drastic reduction in deployment and commissioning time while increasing operational flexibility, paving the way for cost-competitive modular power plants in the near future.
The development team includes SwRI and Hanwha Power Systems. Engineers are combining competitive costs with power on demand, enabling CSP plants to deliver on a brighter future.
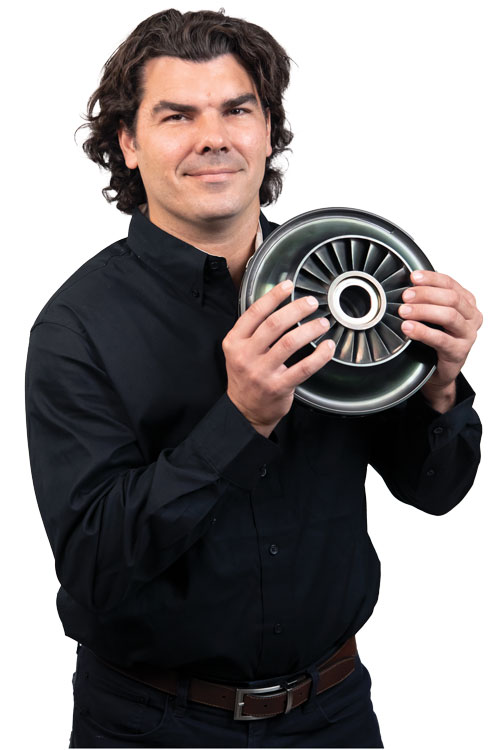
As part of a CSP power plant application, Manager Dr. Jason Wilkes leads a team developing modular, skid-based power cycle technologies including specialized high-temperature, high-pressure sCO2 turbomachinery.
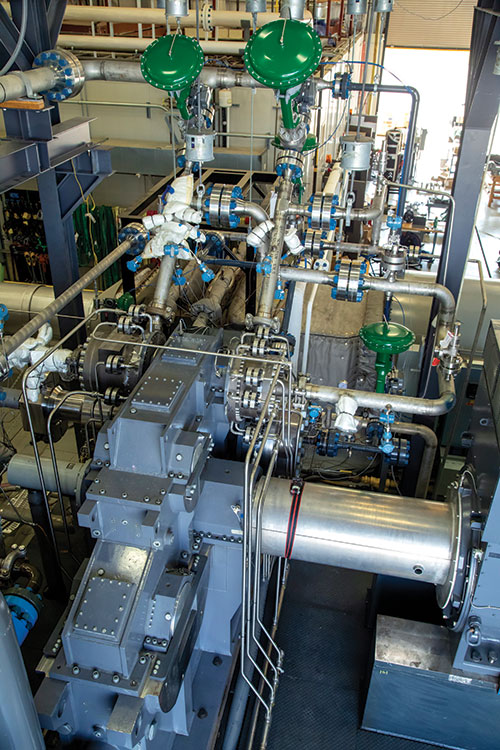
SwRI is developing new technology and processes to create concentrated solar power plants that combine sCO2 power cycles with integrated thermal energy storage.
2. Liquid Air
Aaron RimpelSwRI is leading a DOE project to develop a conceptual design for large-scale, long-duration energy storage with the potential for hundreds of megawatts and tens of gigawatt-hours output. SwRI is working on patent-pending commercial technology to implement liquid air energy storage (LAES), leveraging commercially mature cryogenic refrigeration equipment to liquefy ambient air and store it at near-ambient pressure. Liquid air, stored in large insulated vessels at temperatures as low as -193 C, would experience storage losses of less than 0.1% per day.
Liquid air can be stored when the demand and cost for electricity from conventional or renewable sources is low. When energy demand is high, the liquid air is pumped to higher pressure, vaporized and superheated by a waste heat source such as a gas turbine exhaust stream. The resulting high-pressure gas is expanded through an air turbine, which drives an electrical generator to produce power.
The process uses mature, readily available technologies and draws heavily on established processes and equipment from the power generation and industrial gas sectors. The known costs, performance and life cycles minimize technical risk and promote earlier commercial adoption. In addition, the storage medium, air, is free and ubiquitous, and liquefied air storage can be installed anywhere. Because the exhaust from the power turbine is air, additional energy is produced without further emissions.
Other LAES system concepts utilize additional hot and cold storage subsystems to recuperate energy from the refrigeration (charge) and expansion (discharge) cycles to improve round-trip efficiency. In contrast, the approach being developed on this project simplifies the cycle and focuses on maximizing energy output and revenue per unit of stored liquid air. Because the refrigeration and storage process would utilize low-cost or excess energy from renewables, there is more value in reducing capital costs and increasing power output rather than increasing round-trip efficiency. This conceptual design will optimize the charge, storage and discharge cycles based on cost and performance.
Sponsored by DOE, SwRI is working with Pintail Power to develop its LAES technology to manage the short- and long-term variability associated with renewable resources.
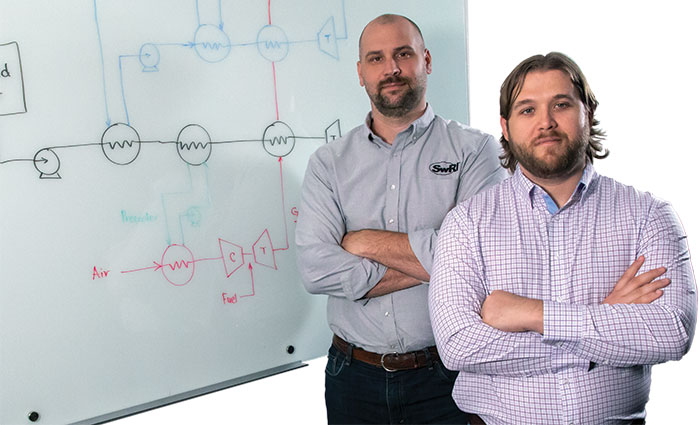
Group Leader Aaron Rimpel (left) and Research Engineer Dr. Owen Pryor are working on a conceptual design for large-scale, long-duration liquid air energy storage with the potential for hundreds of megawatts and tens of gigawatt-hours output.
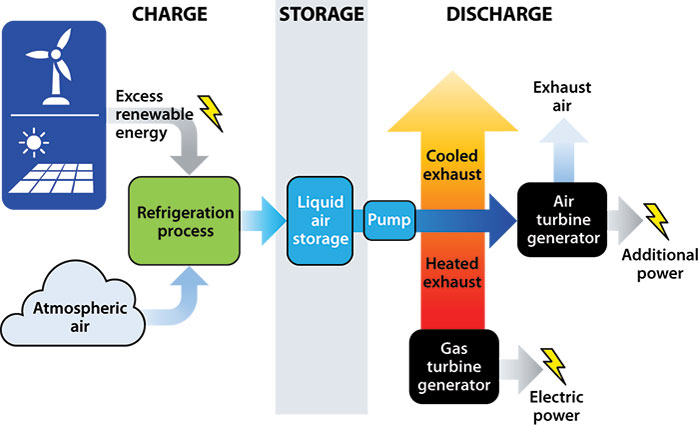
SwRI is researching techniques to store cooled liquid air when the demand and cost for electricity is low. When energy demand is high, waste heat converts the liquid air into high-pressure gas that is expanded through an air turbine to drive an electrical generator and produce additional power.
3. Pumped Heat
Natalie Smith, Ph.D.Pumped Heat Energy Storage (PHES) is another potential long-duration, grid-scale energy storage technology to help maintain grid reliability and security. A PHES system stores energy in hot and cold tanks for later use. Depending on the system, the hot storage media can be molten salt, crushed rock or other materials. The cold storage media is similar to anti-freeze. When excess energy is available, the PHES system runs as a heat pump, similar to any household refrigerator, making the hot store hotter and the cold store colder. Then, when energy demands exceed production, the PHES runs as a heat engine converting the large temperature difference between the hot and cold stores into electricity via a generator. The PHES heat pump and heat engine use compressors, turbines and heat exchangers connected by piping filled with an inert gas, such as air or CO2. PHES offers high potential system performance of more than 60% round-trip efficiency and can store energy for more than 10 hours. PHES is a promising technology offering implementation versatility without geological and geographical constraints.
DETAIL
In energy storage applications, the round-trip efficiency is the storage-to-discharge energy efficiency of the system or the fraction of energy put into storage that can be retrieved as useful energy.
SwRI is conducting two PHES research projects. The first is developing a small-scale PHES facility to demonstrate system operations and control strategies. The SwRI PHES uses air as the working fluid and is configured similarly to a proposed full-scale system. This first-of-a-kind demonstration will also tackle first-implementation challenges and reduce risk for full-scale systems. This PHES demonstration facility is currently under construction on the SwRI campus with testing planned at the end of 2021.
The second project is studying how a full-scale PHES system can improve the environmental and economic performance of a natural gas power plant. The goal is to help balance the diverse power generation on electric grids while improving the reliability and resiliency of the electric system as more intermittent renewables come online. SwRI will use a Texas-based natural gas plant for this feasibility study, where the local energy market is subject to negative pricing at night due to abundant wind energy. In wholesale power markets, negative prices mean a big electricity consumer like a factory or a water treatment plant is paid to consume more power, to meet production and keep the power grid stable. Production and demand must remain balanced to avoid excesses that could damage equipment or cause blackouts.
Establishing the value of using PHES to help balance fossil-fuel energy sources with increased renewable energy capacity will help provide pathways enabling full penetration of renewable resources, like solar and wind, into the electricity grid.
SwRI is collaborating with Malta Inc. and Vistra, a Texas-based integrated retail electricity and power generation company, on these DOE-funded projects.
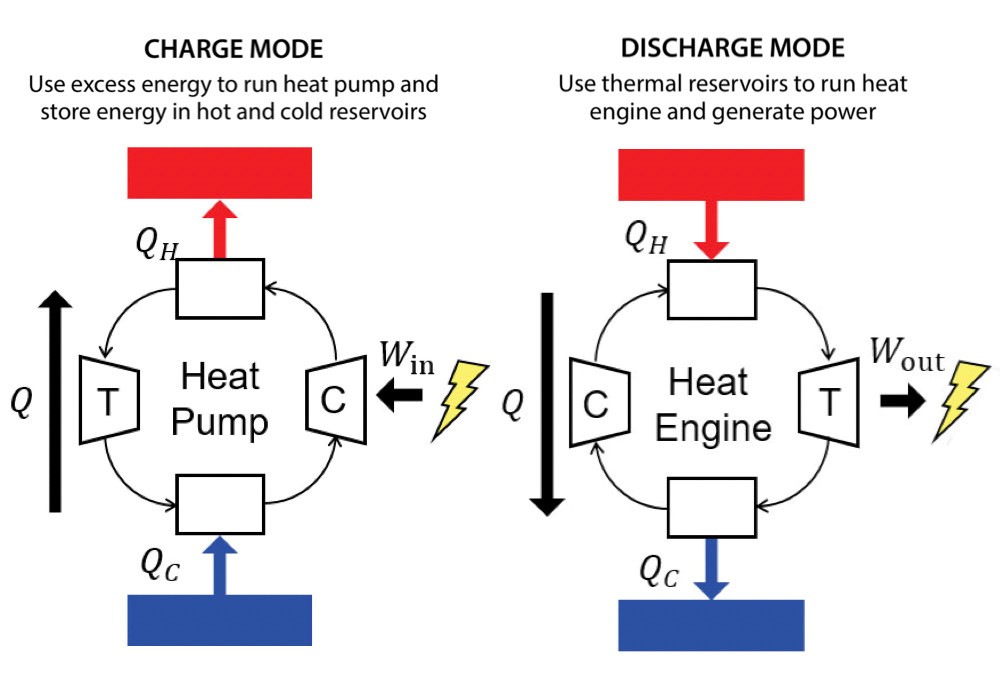
This figure illustrates the basic thermodynamic operation of PHES technology.
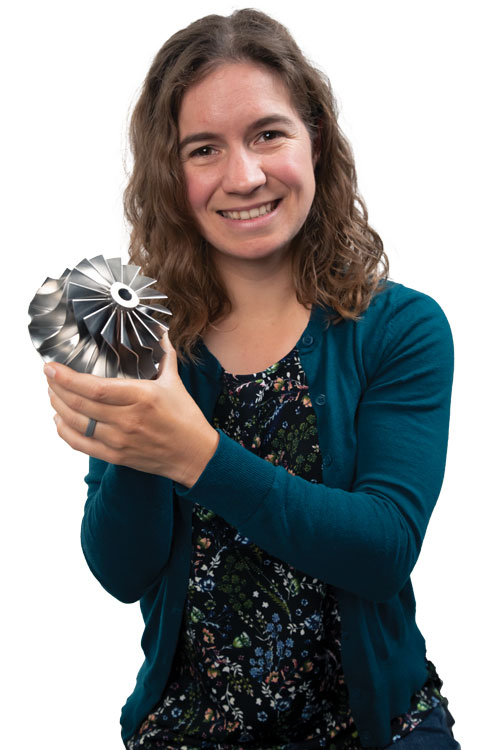
Senior Research Engineer Dr. Natalie Smith is leading a project to develop a small-scale pumped heat energy storage facility, demonstrating system operation and control strategies, addressing first implementation challenges and reducing risk for the full-scale technology. Custom machinery components were developed to enable adequate PHES system performance, including the new discharge turbine impeller shown here.
4. Zero Emissions
Jeff Moore, Ph.D.SwRI is part of a team designing, modeling and optimizing plans for an innovative new zero-emission fossil fuel power plant. With funding from the DOE’s Advanced Research Projects Agency-Energy (ARPA-E), the team will develop the plant, which will incorporate a supercritical carbon dioxide (sCO2) power cycle, renewable energy, oxygen storage and carbon capture.
The electric grid of the future with variable renewable energy sources such as wind and solar power will typically experience surplus energy with low demand, followed by periods of high demand requiring supplemental power from fossil fuel plants. The team proposes using a direct-fired sCO2 power cycle, which uses sCO2 instead of water as a thermal medium and allows far more efficient power generation as well as smaller turbomachinery. The fuel is burned with pure oxygen directly in the working fluid, allowing higher firing temperatures of as much as 1,200 C, approaching those of conventional gas turbines. This process requires an air separation unit (ASU) to provide the power cycle with pure oxygen while extracting CO2 at pressures between 30 and 300 bar, permitting carbon capture while producing no other emissions.
In this power cycle, the ASU requires 13% of the power plant output with an additional 2% required to compress the oxygen. By operating the ASU at higher capacities when excess low-to-zero cost power from alternative energies is available and storing liquid oxygen (LOX) for later use, the power plant achieves higher outputs during periods of peak demand.
Evaporative cooling of the oxygen can reduce the compression power of CO2 in the power cycle and allow additional savings. The ASU equipment is electrically driven, requiring no changes to the power block while producing up to 20% higher power plant output during peak demand. The proposed scheme leverages low-cost power to store a significant amount of energy in the form of LOX, reducing the effective operating costs by up to 20%.
The team includes Air Liquide, 8 Rivers Capital LLC and SoftinWay Inc. and anticipates completing the design stage by the end of 2022.
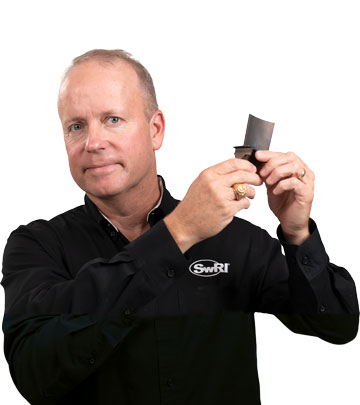
Institute Engineer Dr. Jeff Moore and his team designed this full-scale, internally cooled turbine blade similar to those that will be used in an oxy-fuel direct-fired supercritical carbon dioxide (sCO2) turbine. Each blade generates 600 kW (800 hp) for a total of 450 MW (600,000 hp) of electricity.
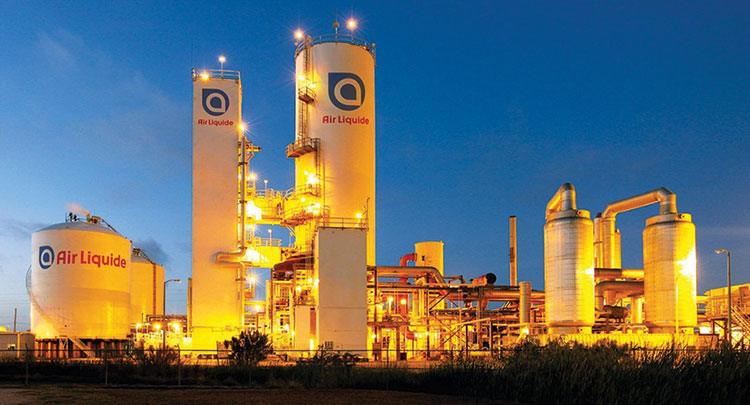
Courtesy of Air Liquide
SwRI is helping develop a next-generation power plant that will incorporate an sCO2 power cycle, renewable energy, oxygen storage and carbon capture.
5. West of the Pecos
Gordon Wittmeyer, Ph.D.If Texas were a country, it would rank fifth in the world in installed wind capacity. Although the Texas grid operator transmits much of the renewable energy generated in western Texas across the state, most wind energy is generated at night when demand and prices are low. Combined with growing solar capacity, the industry has deployed high-ramp-rate gas plants to meet peak evening demand. SwRI proposes using closed-loop pumped storage hydropower (PSH) units to store wind power generated at night, holding it for late afternoon the following day when solar generation drops and reducing the need for more CO2-producing gas peaking plants.
SwRI scientists and engineers have developed technologies and engineering solutions to rapidly deploy low-cost PSH to provide high-volume grid-scale energy storage. In West Texas, storage is needed to stabilize and integrate abundant intermittent wind and solar renewable energy. However, relatively few locations offer both significant topographic relief and flowing rivers to support conventional PSH.
To address this problem, SwRI engineers identified many moderate (300 to 500 foot) to high (650 to 1,000 foot) head sites in West Texas where 50 to 1,000 MW PSH units with four to 16 hours of storage could be constructed. Because the region is semiarid, PSH plants may need to be operated using groundwater; however, groundwater, even saline groundwater, is a precious resource in this region. Instead of groundwater, SwRI scientists determined that waste brines produced by oil and gas operations in the adjacent Permian Basin would be adequate and suitable for PSH units.
Collaborating with the National Renewable Energy Laboratory and Argonne National Laboratory, SwRI has further advanced a low-cost, low-technology modular PSH design that can compete with small grid-scale battery energy storage systems using lithium-ion technology. The team has combined low-weight prefabricated modular steel dams transported by flatbed trailer with low-cost Pumps-As-Turbines (PAT) power units to limit capital expenditures per unit of energy to $100–200/kWh. The team believes the availability of a 10 MW, 100 MWh PSH unit that can be built in a year for $10 million will reinvigorate the PSH industry and can bolster the resilience of the national grid as well as the isolated Texas grid.
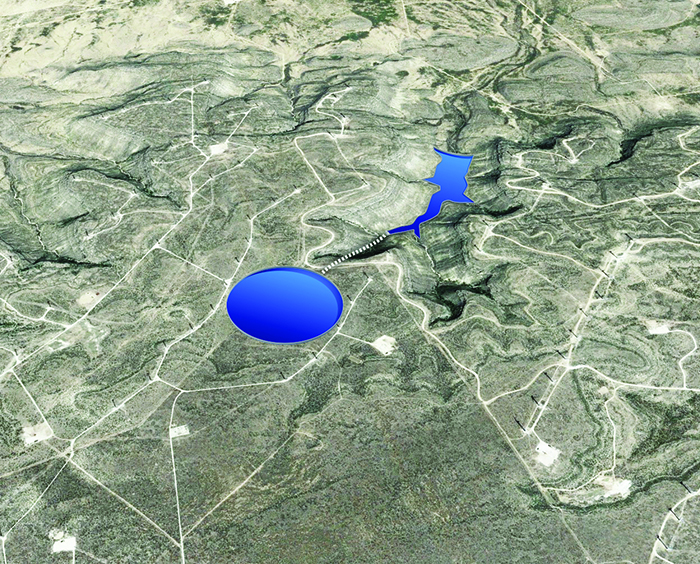
SwRI identified Indian Mesa as a potential PSH site where an upper reservoir (circle) could be built on top of the mesa and lower reservoirs could be built into the canyons below, as illustrated on this Google Earth image. In this PSH application, water is pumped to the high elevation reservoir using low-cost surplus off-peak electric power. During periods of high electrical demand, the stored water is returned to the lower reservoir, driving turbines to produce electric power when demand and prices are highest.
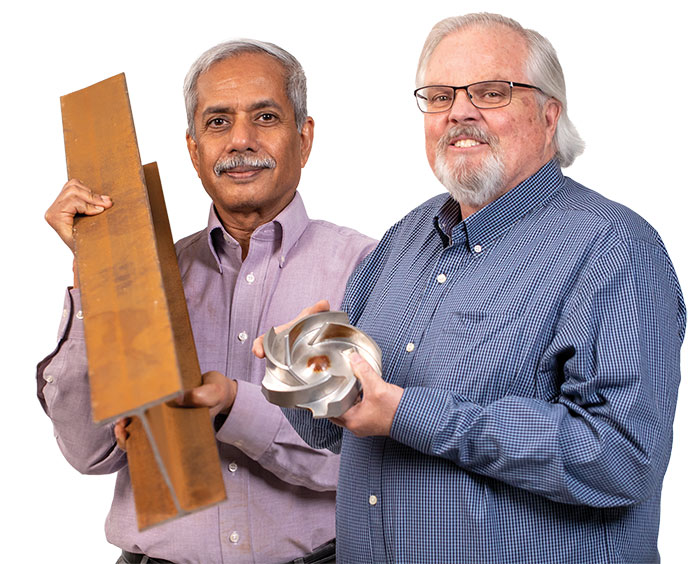
Senior Scientist Dr. Gordon Wittmeyer (right) and Staff Engineer Dr. Biswajit Dasgupta developed an award-winning modular steel dam using standard building materials such as I-beams to accelerate construction of pumped storage. When energy production exceeds demands, PSH systems pump water into a top reservoir using excess power. When power demands exceed production, water is discharged to the lower reservoir, running centrifugal pump impellers such as the one shown in reverse, generating electricity to meet peak demands.
6. Cryogenic Flux Capacitor
Joshua SchmittAlthough it may sound like science fiction, SwRI engineers are working on a hydrogen energy storage solution using a high-density Cryogenic Flux Capacitor (CFC). CFC technology stores fluids at high densities, accepts gaseous hydrogen at ambient conditions and charges up over time. On discharge, controlling heat and regulating the flow of hydrogen gas provides operational flexibility for the total system and allows a wide range of demand loads and duty cycles. The project will demonstrate a CFC storage system using an electrolyzer and assess the inherent flexibility of the coupled system.
The CFC’s cold, dense fluid storage core integrates design features that provide new possibilities for the storage and discharge of energy. This new technology rivals the energy storage capacity of liquefied gases and exceeds battery energy storage, particularly in the case of stored hydrogen. By exploiting a unique attribute of nano-porous materials, aerogel in this case, fluid commodities such as oxygen, hydrogen, methane, etc. can be stored in a molecular surface-adsorbed state. This cryogenic fluid can be stored at low pressure and high densities, on par with liquid, and then quickly released as a gas on demand.
DETAIL
An electrolyzer uses electricity to break water into hydrogen and oxygen in a process called electrolysis, creating hydrogen gas.
For this project, the team will develop a techno-economic assessment and commercialization plan for integration of the technology with various power generation assets. These include using existing natural gas turbines to generate electricity. With incremental conversion and modification of these turbines, asset owners can blend cleaner-burning hydrogen with natural gas in ratios from 15–50%. Higher ratios can be achieved with new turbine designs with an eventual goal of using 100% hydrogen fuel by 2030. The high energy density of CFC-stored hydrogen allows long-term storage, ranging from daily to monthly cycling, which corresponds to approximately 10 to 100 hours, respectively. Many natural gas power plants could accommodate racks of CFC storage units, corresponding electrolysis modules and ancillary equipment. The study would examine a range of storage possibilities for commercial viability. The team is exploring a near-term demonstration at a natural gas combined-cycle power plant. The expected storage size will be 100 megawatt hours (MWh), where the hydrogen will be provided by a 20-megawatt (MW) electrolyzer.
Funded by the Department of Energy, the project team also includes the University of Central Florida, NASA Kennedy Space Center, Air Liquide and Turbine Technology Services.
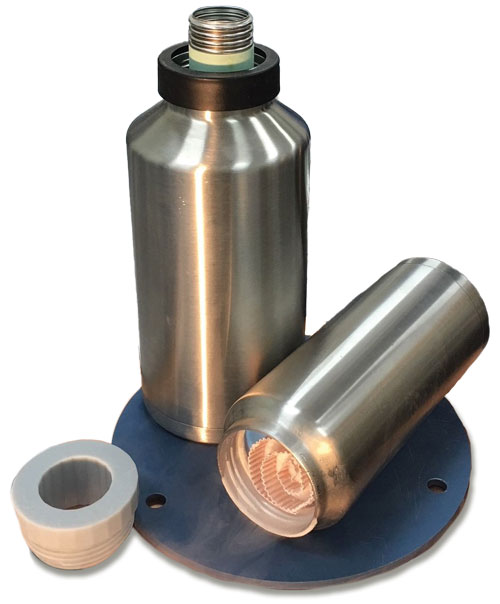
Courtesy of NASA-KSC
SwRI is studying how NASA-developed cryogenic flux capacitor technology could be used to store 100 megawatt hours of energy.
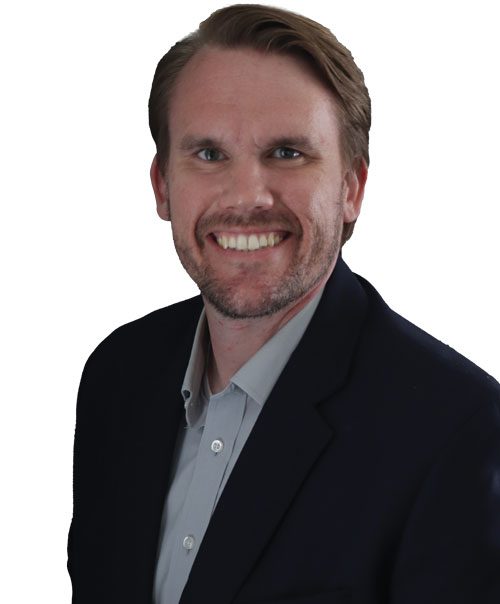
Senior Research Engineer Joshua Schmitt is leading a hydrogen energy storage project, investigating using a high-density cryogenic flux capacitor to store energy as super-cooled fluids at high densities.
Questions about these six projects or Machinery? Contact Tim Allison at +1 210 522 3561.
The information, data or work presented herein was funded in part by the U.S. Department of Energy, under award numbers DE-FE0032002, DE-AR0001313, DE-FE0032003, DE-AR0001018, DE-EE0007114 and DE-FE0032031. The views and opinions of authors expressed herein do not necessarily state or reflect those of the United States Government or any agency thereof.